kimia dengan kadar tertentu lingkungan (3)
Bebas
876
0
0
Teks penuh
Gambar

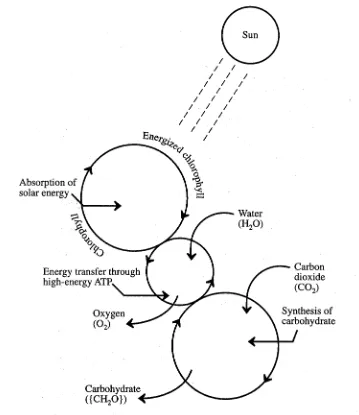
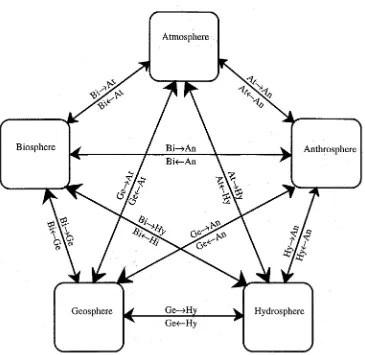

+7
Garis besar
Dokumen terkait